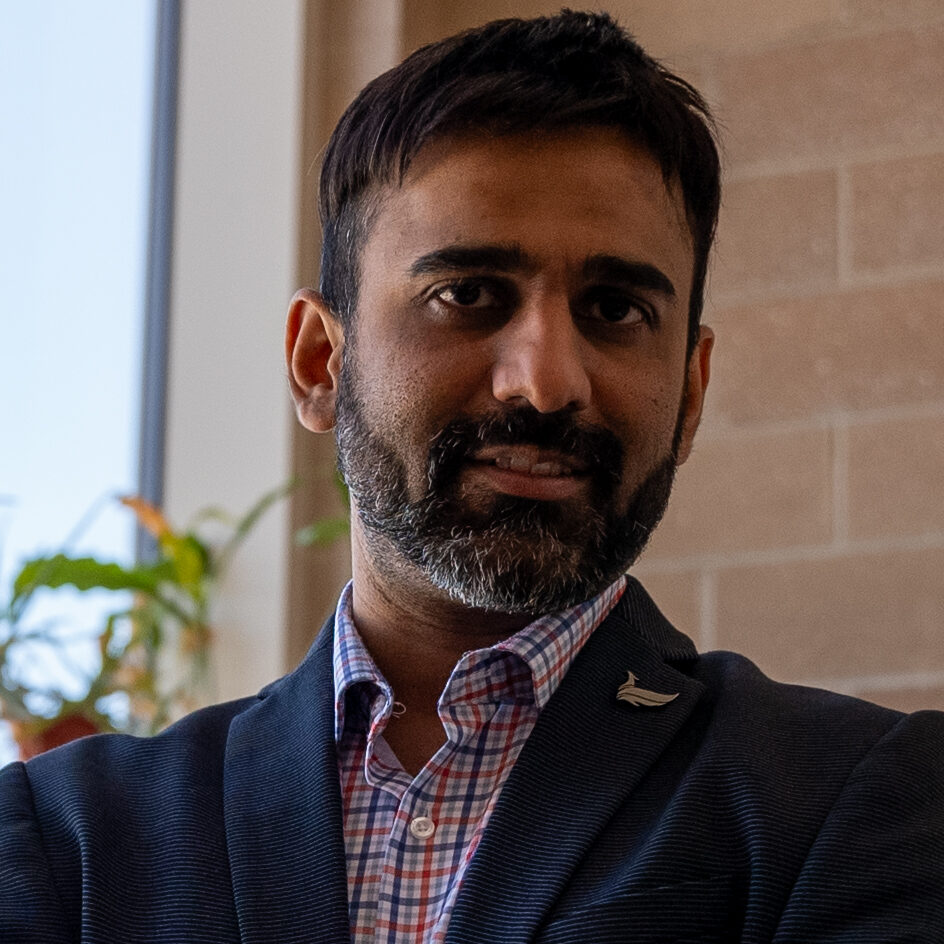
Complex alloys incorporate multiple metals or other principal elements, giving them desirable properties for a range of modern applications in aerospace and beyond– but their complex nature does not necessarily mean they do not rust or fail over time. A new study provides a deeper understanding of how oxidation occurs in these complex alloys. By applying this understanding, the oxidation behavior and lifetime of similar alloys can now be predicted for technologies.

The term “complex alloy” generally refers to alloys that are elementally complex – meaning that they incorporate multiple elements. This study focused specifically on high-entropy alloys, which consist of five or more metals in approximately equal amounts. High-entropy alloys are of interest because they can form simple stable crystal structures that give the alloy desirable mechanical properties over a wide range of extremities like temperature and pressure. But whether you plan to use a high-entropy alloy in high-strength structures or in industrial catalysis, you need to know how the material will degrade or fail over time.
“One common way that materials degrade is oxidation – materials interact with oxygen and break down,” says Bharat Gwalani, corresponding author of a paper on the work and an assistant professor of materials science and engineering at North Carolina State University. “But predicting oxidation for complex alloys, such as high-entropy alloys, is complicated. That’s because all of the elements in the alloy interact differently with both oxygen and each other – particularly at elevated temperatures. This work sheds light on exactly how oxidation takes place in complex alloys and will inform both the development of future alloys and how we can use those alloys in real-world applications.”

For this study, researchers looked at an alloy made up of equal amounts of cobalt, chromium, iron, nickel, and manganese (CoCrFeNiMn) in a controlled oxygen environment. These types of alloys are being researched as cost-effective replacements for the more expensive nickel and cobalt-based ones used in high-temperature applications.
Specifically, the researchers used both bulk and microscale samples of CoCrFeNiMn that were exposed to oxygen at 400 degrees Celsius. The elevated temperature was important because higher temperatures make the material more susceptible to oxidation and therefore accelerate this process. By using a variety of techniques – including atomic resolution transmission electron microscopy, X-ray absorption near edge spectroscopy, and in situ atom probe tomography – the researchers were able to gain a deeper understanding of how oxidation takes place in complex alloys and how the structure of oxides at the surface of these alloys evolves over time. The team also applied a new method for the first time to high entropy alloys, namely grazing-incidence wide-angle X-ray scattering (GIWAXS). This surface-sensitive structural measurement was performed at Brookhaven National Laboratory in collaboration with Prof. Aram Amassian and Dr Ruipeng Li.
“This work sheds light on the mechanisms of oxidation in complex alloys at the atomic scale. What’s more, our findings are not limited to oxidation in CoCrFeNiMn, or even oxidation in high-entropy alloys. By understanding the fundamental mechanisms involved, this work gives us a deeper understanding of oxidation across many complex alloys. And this is just the beginning.”
Prof. Martin Thuo
The work on the surface organization of oxides has implications beyond material degradation and has been applied by one of the NC state collaborators, Prof. Martin Thuo, to engineer metastability in the bulk leading to new technologies like heat-free solders or revealing new material states.
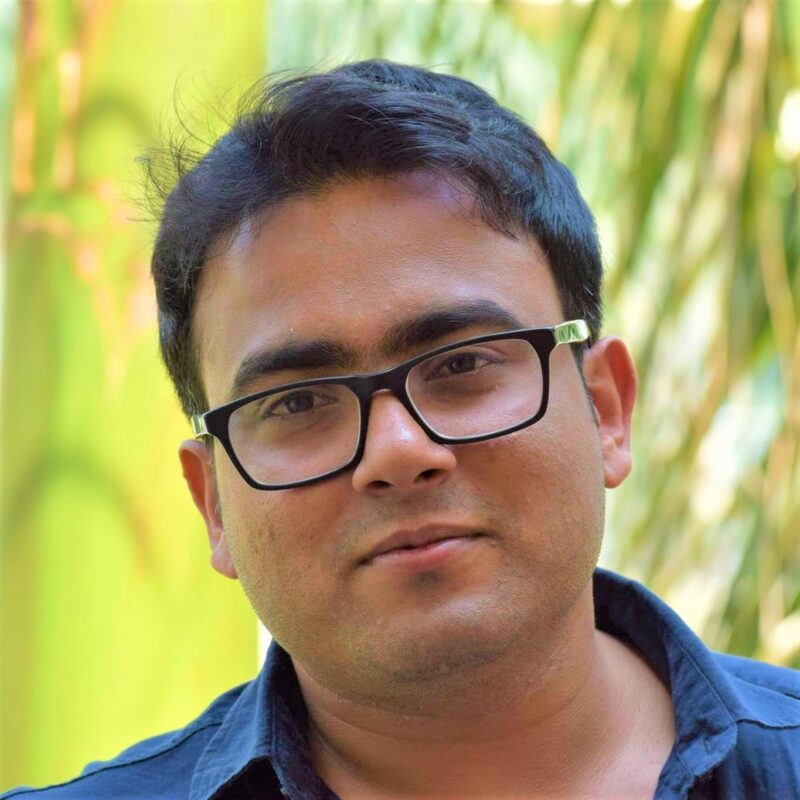
Now, this multidisciplinary research team is working on developing high throughput techniques for theorizing, manufacturing and rapidly characterizing alloys for suitability to various extremities. This will help design next-generation materials with enhanced resistance to extreme environments, suitable for aerospace and nuclear power industries.
The paper, “Mechanistic Understanding of Speciated Oxide Growth in High Entropy Alloys,” is published in the open-access journal Nature Communications. Arun Devaraj of Pacific Northwest National Laboratory (PNNL) is the co-corresponding author of the paper. The paper was co-authored by Andrew Martin and Aniruddha Malakar, postdoctoral researchers at NC State; Boyu Guo, a Ph.D. student at NC State; Elizabeth Kautz, an assistant professor of nuclear engineering at NC State who has a joint appointment with PNNL; Martin Thuo and Aram Amassian, professors of materials science and engineering at NC State; S.V. Lambeets, Matthew Olszta, Anil Krishna Battu and Thevuthasan Suntharampillai of PNNL; Feipeng Yang and Jinghua Guo of Lawrence Berkeley National Laboratory; and Ruipeng Li of Brookhaven National Laboratories.
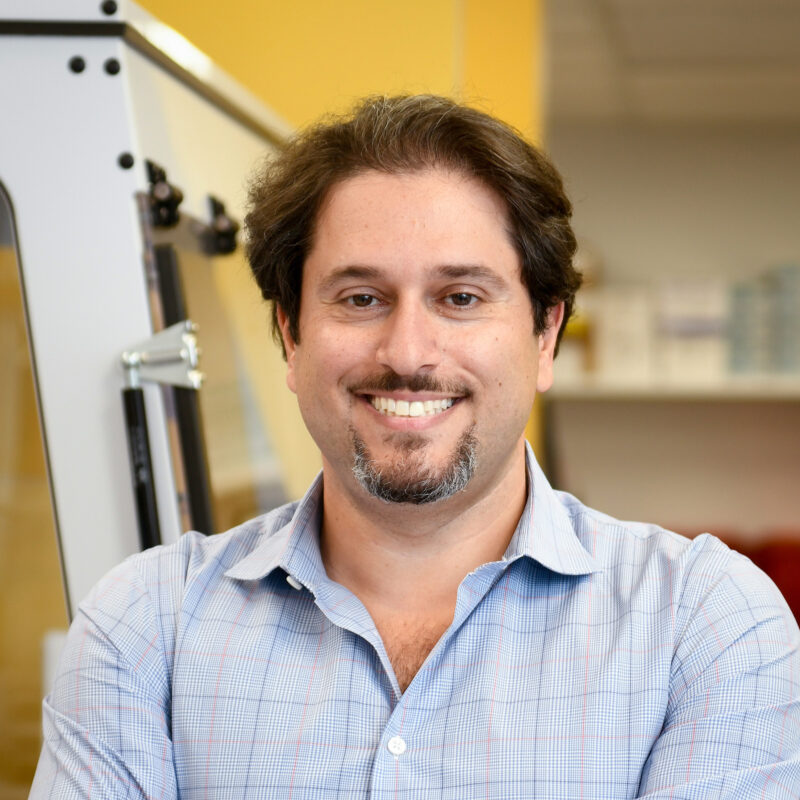
This work was done with support from the Office of Naval Research, under grant N00014-23-1-2758; the Department of Energy, Office of Science, Basic Energy Sciences, Materials Sciences and Engineering Division as part of the Early Career Research Program under grant FWP 76052; the PNNL; and the Advanced Light Source at Lawrence Berkeley National Laboratory.
-shipman-

Note to Editors: The study abstract follows.
“Mechanistic Understanding of Speciated Oxide Growth in High Entropy Alloys”
Authors: Bharat Gwalani, Andrew Martin, Boyu Guo, Aniruddha Malakar, Aram Amassian and Martin Thuo, North Carolina State University; Elizabeth Kautz, Pacific Northwest National Laboratory and North Carolina State University; S.V. Lambeets, Matthew Olszta, Anil Krishna Battu, Thevuthasan Suntharampillai and Arun Devaraj, Pacific Northwest National Laboratory; Feipeng Yang and Jinghua Guo, Lawrence Berkeley National Laboratory; and Ruipeng Li, Brookhaven National Laboratories.
Published: June 12, 2024, Nature Communications
DOI: 10.1038/s41467-024-49243-8
Abstract: Complex multi-element alloys are gaining prominence for structural applications, supplementing steels, and superalloys. Understanding the impact of each element on alloy surfaces due to oxidation is vital in maintaining material integrity. This study investigates oxidation mechanisms in these alloys using a model five-element equiatomic CoCrFeNiMn alloy, in a controlled oxygen environment. The oxidation-induced surface changes correlate with each element’s interactive tendencies with the environment, guided by thermodynamics. Initial oxidation stages follow atomic size and redox potential, with the latter becoming dominant over time, causing composition inversion. The study employs in-situ atom probe tomography, transmission electron microscopy, and X-ray absorption near-edge structure techniques to elucidate the oxidation process and surface oxide structure evolution. Our findings deconvolute the mechanism for compositional and structural changes in the oxide film and will pave the way for a predictive design of complex alloys with improved resistance to oxidation under extreme conditions.